Power
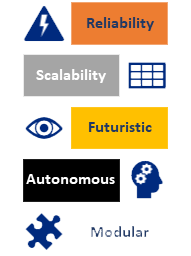
The power system, comprising PV modules, inverter, battery, and distribution system is arguably the most important mechanical system of the house. Apart from providing power to meet the demands of the other subsystems in a seamless and uninterrupted manner – particularly for critical systems – the power system must also be capable of regulating optimal battery charge levels in both the primary and electric vehicles to enable flexible choices in when to purchase or sell power to the grid.
The power system can be broadly divided into three primary operations: generation (PV modules, optimizers, inverter, battery), distribution (panels and wiring), and monitoring (power generation and consumption monitoring, system performance assessment). Given this wide range a equipment combinations and choices, a rational means of selecting the best choice for react was developed to compare reliability, scalability, use of state-of-the-art technology, autonomy, and modularity to compare different design alternatives. What was revealed was that much of the decision process was driven by the inverter configuration. For example string inverters, while well-known, extensively tested, and established in the marketplace, fall short in terms of modularity, autonomy, and efficiency when the string is partly shaded. More importantly, without significant modifications, standard string inverter-based systems are incapable of rapidly shutting down in some emergency situations. Micro-inverter systems address many of the safety concerns associated with string inverters. While they are capable of module-level control, they can suffer when used in long strings, such as those used on each wing of reACT. And like string inverter systems, the power output of micro-inverters must be rectified when battery storage is used, reducing overall system flexibility and performance
What we have produced is a virtual house that can be placed anywhere in the US, as well as most places in the world. Since October last year and shortly after local midnight every day since, a sequence of Python scripts automatically read the weather reports for College Park and the 2017 Solar Decathlon competition site in Denver and predicts the performance of Team Maryland's virtual reACT houses over that day (reactvirtual.eng.umd.edu). Since the onset of this project, we have been accumulating predicted performance data in terms of solar power produced, power consumed by household events, such as charging the car early each morning and making dinner in the evening, and then translating that into the instantaneous profits and costs (see Figure 2 below). The plots illustrate the day's performance - even on the partly cloudy day shown, our virtual house predicts a significant net-positive energy behavior and a tiny virtual profit for the virtual occupants.
Our ultimate power system design choice was based on using module-level DC-DC optimizers. This design choice satisfied all of our selection criterion by allowing for modular-level control and safety in emergencies and loss of grid power, sophisticated cloud-based module performance monitoring capabilities, automatic PV array optimization that is perfectly suited to the differing orientation of reACT’s two PV arrays, and direct DC charging of our primary house battery system. Specifically, we chose to use SolarEdge’s StorEdgeTM inverter and optimizer, a 9.8 kWh LG Chem lithium-ion battery, and two PV arrays each consisting of fourteen SunPower SPR-X21-335 PV modules producing 9.4 kW at maximum power conditions.
An important aspect of this configuration is the ability to program the battery charging policy into a set of distinct operating modes, such as always maintaining battery change when battery backup is a priority, or purchasing and selling power based on the power rate schedule. This ability, in conjunction with virtual reACT’s ability to predict and select the optimal mode, represents a key element in out home automation technology.